Antimatter atoms held captive by physicists
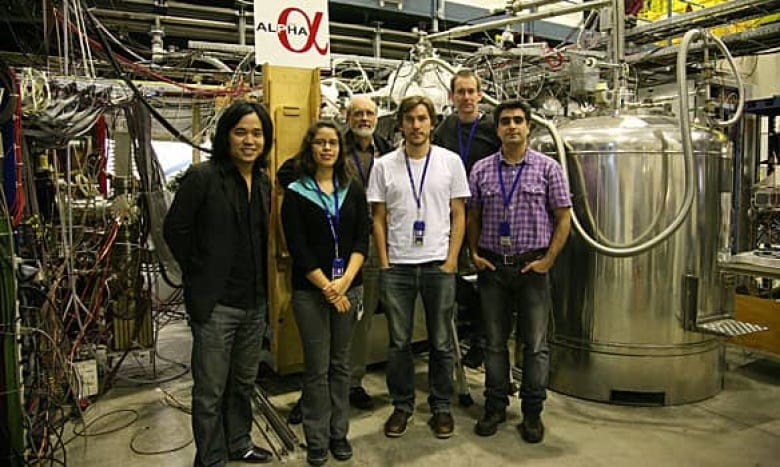
For the first time, antimatter atoms have been caged and kept in existence long enough to be probed by scientific instruments.
"We're very excited about the fact that we can actually now trap antimatter atoms long enough to study their properties and see if they're very different from matter," said Makoto Fujiwara, who led the Canadian contribution to ALPHA, the international collaboration that made the discovery.
The results, published online Wednesday in Nature, mean scientists are closer to solving the mystery of what happened to most of the antimatter in the universe, Fujiwara said.
Antimatter is produced in equal quantities with matter when energy is converted into mass — this happens in particle colliders and is believed to have happened during the Big Bang at the beginning of the universe. That's why physicists are puzzled about why there is no longer any significant amount of antimatter in the universe.
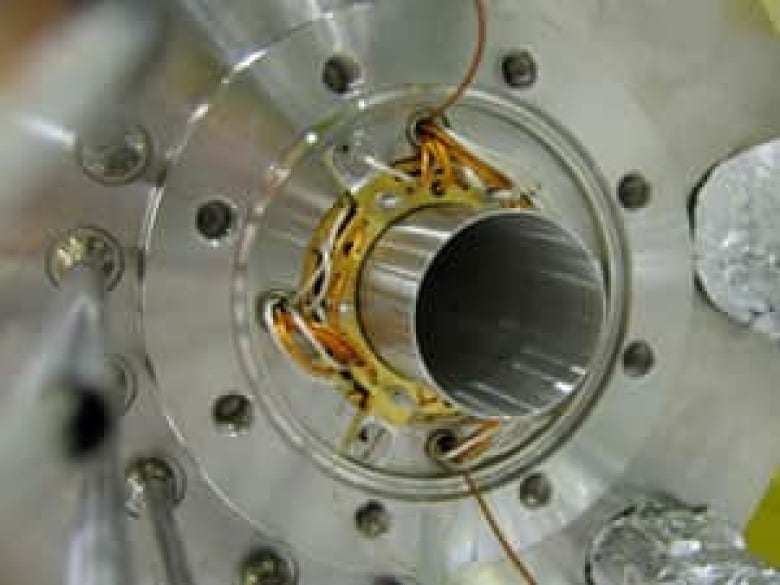
Scientists would like to be able to study antimatter to figure out how it is different from matter, as that might provide clues about the apparent disappearance of antimatter. But there's a problem — when antimatter and matter encounter each other, they both get annihilated, producing pure energy.
Because our world is made of matter, antimatter atoms produced artificially until now have lasted just millionths of a second before hitting the matter walls of their container and getting annihilated.
The latest study, which took place at the CERN Laboratory near Geneva, Switzerland, has found a way to trap atoms of antihydrogen away from the walls of their container to prevent them from getting annihilated for nearly one-10th of a second.
Antimatter basics
Particles of antimatter have the same mass but opposite charge to their corresponding matter particle. The antiparticle of a proton is an antiproton and has a negative charge. The antiparticle of an electron — an antielectron, usually called a positron — has a positive charge.
Audio
York University physicist Scott Menary, who took part in the research, will be interviewed on Quirks & Quarks on Saturday, Nov. 20, at noon on CBC Radio One.
Because these antiparticles carry a charge, they can be contained in electric fields for days and used in experiments. Positrons are used in PET scanners, a medical device used to create three-dimensional images of cancerous tumours and to detect the spread of cancer.
Positrons are produced naturally during the decay of certain radioactive elements, such as carbon-11. They can also be produced in the lab, for example, by blasting gold with high-powered lasers.
Antiprotons are produced in particle accelerators and can also be detected in space when cosmic rays collide with the Earth's atmosphere.
Antihydrogen is made by mixing antiprotons and positrons. The resulting antimatter atoms have a neutral charge, so they can't be trapped in an electric field.
'Tiny, tiny magnet'
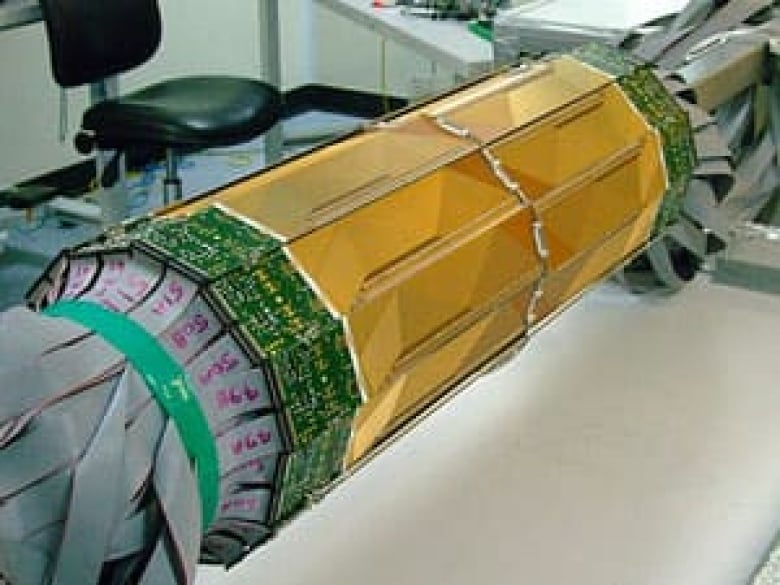
The magnet is so small that even using an extremely powerful magnetic field generated by a superconducting magnet, the researchers could only generate a small magnetic force on the antihydrogen atoms. But that was enough to create a magnetic trap that could hold some of them.
"You can only capture really the slowest ones," Fujiwara said.
To improve their chances, the researchers mixed the antiprotons and positrons "very gently" in a very cold vacuum so that they were hardly moving at all.
After trapping the antimatter atoms for some time, the researchers turned off the magnetic field, letting the atoms out of the trap. The atoms immediately hit matter and were annihilated, producing detectable signals. That is how the researchers knew they had successfully trapped 38 of the atoms.
How the magnetic trap works
The magnetic trap can be imagined as a shallow dish where spherical atoms of antimatter are rolling around like balls, and the magnetic force is analogous to gravity. If the balls are moving too quickly, they will jump over the lip of the dish, escape, and hit the matter around them. But if they are moving very slowly, then they will roll back toward the centre of the dish whenever they get too close to the edge.
When the magnetic field is turned off, the dish loses its concave shape and becomes completely flat. Then, there is nothing to keep the atoms in the dish and even the slowest ones will roll right over the edge.
This is a figurative description of the forces experienced by the antimatter atoms. It doesn't describe their physical environment, which is a metal container under vacuum.
The electronics for the annihilation detector were built by the Canadian members of ALPHA, Fujiwara said.
Richard Hydomako, a PhD student working with Prof. Rob Thompson at the University of Calgary and Prof. Scott Menary at York University played a crucial role in the data analysis, TRIUMF reported.
The next step for the collaboration is to conduct experiments on the trapped antimatter atoms.
University of British Columbia physicist Walter Hardy and Michael Hayden, a physicist at Simon Fraser University, are currently working on a method to find out what colour light the antihydrogen shines when it is hit with microwaves, Fujiwara said.
The colours shone by hydrogen atoms are well known and researchers are interested in knowing how similar antihydrogen will be.
"It's going to be a long effort again. This is a very challenging goal," Fujiwara said.
But researchers are "very excited" about the possibilities.
Forty-two physicists from 15 institutions around the world contributed to the research, including 13 from Canada. Other participating countries were Brazil, Denmark, Israel, Japan, Sweden, the U.K., and the United States.
Canadian funding for the research included contributions from the federal government and the Alberta and Quebec governments, as well as the Killam Trusts.